akelm
100 µW
Most of us have seen plots produced by the Crystalyte motor simulator at http://ebikes.ca/simulator/.
These plots show graphs of motor torque, output power and efficiency as functions of speed, for a fixed throttle setting. While such plots are very useful for understanding motor performance, the behaviour of an e-bike depends on other factors, such as air drag, rolling resistance and power needed for climbing slopes. A common way to try to bring these factors into consideration is to plot a curve of power requirements as a function of speed. This gives rise to a plot like the following:
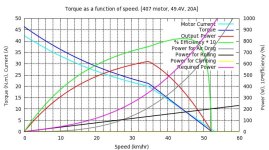
The motor torque, power and efficiency curves in the above plot are calculated based on the model used by the ebikes.ca simulator, and have been validated against it (http://docs.google.com/View?docID=ddsfhm9d_30cn55cz7v#Part3 ).
The calculation of the power requirement curves
(http://docs.google.com/View?docID=ddsfhm9d_30cn55cz7v#Part4)
depends on the choice of a number parameters, so the power requirements should only be regarded as a representative example. (The actual choice of parameters for this plot is listed beside the next plot, which uses the same values. Wheel size is 26â€Â).
The point where the power requirement curve matches the motor output power curve indicates how fast the e-bike will go under these circumstances: 40.5 km/hr. This graph doesn’t tell us how the bike performs on different slopes, against different winds, or with different throttle settings. As it is natural to try to drive an e-bike in such a way as to maintain a target speed through various terrain and wind conditions, a fixed speed plot would be useful. The following graph shows such a plot, for cruising (with the help of a CycleAnalyst, say) at the legal limit of 32 km/hr.
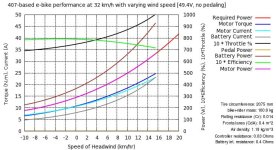
This graph shows, for example, that to maintain 32 km/hr without wind or hill requires 7.6A of battery current. The strongest wind we can maintain this speed against is about 15.5 km/hr, which corresponds to 100% throttle and a battery current draw of about 23A. If a 500W output power limit is to be observed (as required in Canada), only 10 km/hr wind can be countered at this speed, and that requires 15A of battery current. This fixed speed plot provides quite a bit of information regarding performance of this e-bike. A separate plot (not shown),with 150W of pedal power added, shows that without wind, only 3.4A of battery current is required to maintain 32 km/hr.
What if we are interested in hill climbing? The following graph shows the same bike configuration, without wind but on various hill grades and with 150W of pedal power added.
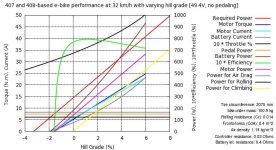
The steepest hill this e-bike can maintain 32 km/hr on (with 150W pedaling power) is a 6% grade, but that requires 23A of battery current. Keeping the 500W output motor power limit, only a 4% grade can be handled at 32 km/hr, and that requires 15A of battery current.
While the above plots are quite useful for the range of conditions under which the target speed of 32 km/hr can be maintained, they don’t tell how much the e-bike would slow down for greater headwind speeds or steeper grades. The following ‘contour plots’ provide such information, as they show the combinations of ground speed and wind speed (or ground speed and hill grade) that can be handled for specific battery current levels. The thick red curve on the plots show the 500W output power limit, and the magenta curve shows the 100% throttle line, which is a limiting factor at higher velocities.
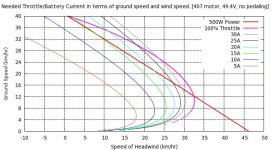
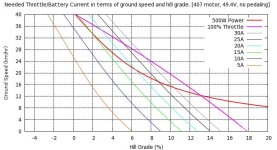
Further analysis and discussion of such plots and the theory behind them, along with many other examples, is found in a document I have placed on Google documents:
http://docs.google.com/View?docID=ddsfhm9d_30cn55cz7v
In particular, it has a download link so that you can download the Gnuplot scripts used to produce such plots, in order to experiment with them yourself. I hope that some of you will, and that these new plots will provide added insight into e-bike performance and behaviour.
These plots show graphs of motor torque, output power and efficiency as functions of speed, for a fixed throttle setting. While such plots are very useful for understanding motor performance, the behaviour of an e-bike depends on other factors, such as air drag, rolling resistance and power needed for climbing slopes. A common way to try to bring these factors into consideration is to plot a curve of power requirements as a function of speed. This gives rise to a plot like the following:
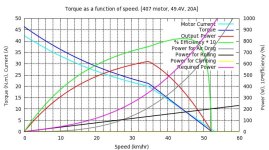
The motor torque, power and efficiency curves in the above plot are calculated based on the model used by the ebikes.ca simulator, and have been validated against it (http://docs.google.com/View?docID=ddsfhm9d_30cn55cz7v#Part3 ).
The calculation of the power requirement curves
(http://docs.google.com/View?docID=ddsfhm9d_30cn55cz7v#Part4)
depends on the choice of a number parameters, so the power requirements should only be regarded as a representative example. (The actual choice of parameters for this plot is listed beside the next plot, which uses the same values. Wheel size is 26â€Â).
The point where the power requirement curve matches the motor output power curve indicates how fast the e-bike will go under these circumstances: 40.5 km/hr. This graph doesn’t tell us how the bike performs on different slopes, against different winds, or with different throttle settings. As it is natural to try to drive an e-bike in such a way as to maintain a target speed through various terrain and wind conditions, a fixed speed plot would be useful. The following graph shows such a plot, for cruising (with the help of a CycleAnalyst, say) at the legal limit of 32 km/hr.
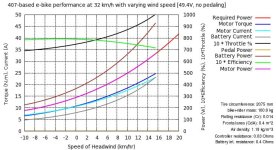
This graph shows, for example, that to maintain 32 km/hr without wind or hill requires 7.6A of battery current. The strongest wind we can maintain this speed against is about 15.5 km/hr, which corresponds to 100% throttle and a battery current draw of about 23A. If a 500W output power limit is to be observed (as required in Canada), only 10 km/hr wind can be countered at this speed, and that requires 15A of battery current. This fixed speed plot provides quite a bit of information regarding performance of this e-bike. A separate plot (not shown),with 150W of pedal power added, shows that without wind, only 3.4A of battery current is required to maintain 32 km/hr.
What if we are interested in hill climbing? The following graph shows the same bike configuration, without wind but on various hill grades and with 150W of pedal power added.
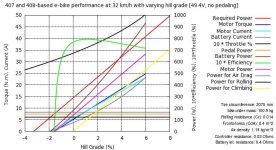
The steepest hill this e-bike can maintain 32 km/hr on (with 150W pedaling power) is a 6% grade, but that requires 23A of battery current. Keeping the 500W output motor power limit, only a 4% grade can be handled at 32 km/hr, and that requires 15A of battery current.
While the above plots are quite useful for the range of conditions under which the target speed of 32 km/hr can be maintained, they don’t tell how much the e-bike would slow down for greater headwind speeds or steeper grades. The following ‘contour plots’ provide such information, as they show the combinations of ground speed and wind speed (or ground speed and hill grade) that can be handled for specific battery current levels. The thick red curve on the plots show the 500W output power limit, and the magenta curve shows the 100% throttle line, which is a limiting factor at higher velocities.
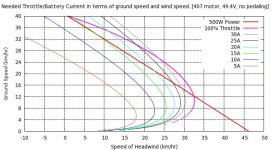
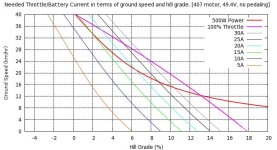
Further analysis and discussion of such plots and the theory behind them, along with many other examples, is found in a document I have placed on Google documents:
http://docs.google.com/View?docID=ddsfhm9d_30cn55cz7v
In particular, it has a download link so that you can download the Gnuplot scripts used to produce such plots, in order to experiment with them yourself. I hope that some of you will, and that these new plots will provide added insight into e-bike performance and behaviour.